Understanding Theoretical Yield: Essential Guidelines for 2025
Theoretical yield is a key concept in chemistry, particularly when it comes to evaluating the efficiency of chemical reactions. Understanding how to calculate theoretical yield helps scientists estimate how much product can be derived from specific amounts of reactants, laying the groundwork for practical applications in research and industry alike. Knowledge of this metric not only aids in the assessment of reaction efficiency but also plays a crucial role in optimizing reaction conditions.
In this article, we will delve into the theoretical yield formula, discuss the importance of theoretical yield in chemistry, and explore the various factors influencing reaction yields. Whether you're a student eager to master stoichiometric calculations or an experienced chemist looking to refine your methods, this guide will provide essential insights into calculating theoretical yield effectively.
Key takeaways include the distinction between theoretical yield and experimental yield, the significance of limiting and excess reactants, and practical examples illustrating the calculation methods. Let's embark on this comprehensive journey into the world of theoretical yield.
Calculating Theoretical Yield: The Basics
To effectively calculate theoretical yield, one must first grasp the basic definitions and concepts related to yield. Theoretical yield refers to the maximum amount of product that could theoretically be produced from given quantities of reactants in a chemical reaction. This value is determined through stoichiometric calculations based on the balanced chemical equation.
Before performing yield calculations, it’s crucial to balance the chemical equation correctly. This is essential as the balanced equation provides the molar ratios needed for the calculations. Once the equation is balanced, identifying the limiting reactant is the next key step. The limiting reactant is the substance that will be fully consumed first, thus dictating the amount of product that can be formed.
Here, we'll look at a typical example: If the balanced equation shows that 2 moles of reactant A react with 1 mole of reactant B to produce 2 moles of product C, calculating the theoretical yield will hinge on knowing the initial amounts of A and B.
The Theoretical Yield Formula Explained
The theoretical yield formula can be summarized as follows:
Theoretical Yield (grams) = (moles of limiting reactant) × (molar mass of product)
In practice, this means you will first calculate the moles of the limiting reactant based on its initial mass and molar mass. Multiply the moles of the limiting reactant by the stoichiometric coefficients from the balanced chemical equation to find the number of moles of product formed. Finally, converting moles of the product to grams by using its molar mass gives you the theoretical yield. This methodical approach ensures accurate calculations of yield, vital for achieving the desired outcomes in chemical processes.
Importance of Theoretical Yield in Chemistry
Theoretical yield is significant for multiple reasons. Primarily, it acts as a benchmark for evaluating the efficiency of reactions. By comparing the theoretical yield with the actual yield obtained from an experiment, chemists can assess how effectively a reaction proceeds. This comparison allows for an evaluation of not only the efficiency but also the practicality of the reaction conditions used.
In addition, understanding theoretical yield helps researchers optimize chemical reactions. Factors such as temperature, pressure, and the presence of catalysts can impact the theoretical yield. By manipulating these factors based on the theoretical yield calculations, chemists can steer their processes towards greater efficiency and yield.
Comparing Ideal Yield vs. Theoretical Yield
When discussing yield, it’s important to distinguish between ideal yield and theoretical yield. While theoretical yield is calculated based on stoichiometric relationships in balanced equations, ideal yield refers to the yield attained under optimal conditions without any losses. In real-world laboratory settings, the actual yield often falls short of both due to variable conditions and side reactions, illustrating the phenomenon of yield loss.
Moreover, the ideal yield represents the best-case scenario. Understanding these differences can help chemists and students alike comprehend where discrepancies in yield may arise in practical applications. It aids in forming strategies to mitigate these losses and improve overall reaction outcomes.
Experimental Yield vs. Theoretical Yield: Key Differences
When comparing experimental yield and theoretical yield, the key difference lies in their definitions: experimental yield refers to the amount of product actually obtained from a reaction, while theoretical yield is the amount predicted based on stoichiometric calculations. Understanding this distinction is crucial for evaluating the success of a chemical process.
To calculate the yield percentage, the following formula is used:
Yield Percentage = (Experimental Yield / Theoretical Yield) × 100%
Monitoring and comparing these yields can provide insights into the efficiency of the reaction as well as guide necessary adjustments to optimize the outcomes, paving the way for higher yield percentages and better resource utilization.
Factors Affecting Theoretical Yield Calculation
Several factors can influence the yield of a chemical reaction. The most prominent among these are:
- Limiting Reactant: This reactant determines the maximum amount of product that can be formed. Identifying it is crucial to accurate yield calculations.
- Reaction Conditions: Temperature, pressure, and catalysts can significantly alter how a reaction proceeds, impacting the theoretical yield.
- Side Reactions: Uncontrolled side reactions can consume reactants, thus reducing the amount of product formed.
By understanding these factors, chemists can better predict and calculate theoretical yield, tailoring their experiments for optimal results. Adjusting reaction conditions or reevaluating reactant mixtures can lead to significant improvements in yield.
Laboratory Techniques for Optimizing Theoretical Yield
Optimizing theoretical yield involves using various laboratory techniques that can enhance reaction efficiency. Key strategies include:
- Careful Measurement: Accurately measuring reactants decreases excess waste and improves yield calculations.
- Controlling Reaction Conditions: Fine-tuning temperature and pressure can lead to more favorable reaction pathways, thus enhancing theoretical yield.
- Utilizing Catalysts: Introducing catalysts can accelerate reactions and minimize side products, contributing positively to yield.
By employing these techniques, chemists can refine their methods and achieve higher theoretical yield through well-planned experimental designs and procedural rigor.
Theoretical Yield Examples to Illustrate Calculations
To solidify our understanding of how to find theoretical yield, let’s consider a simple example:
In a reaction where hydrogen gas reacts with oxygen gas to form water, we start with 3 moles of hydrogen and 2 moles of oxygen:
- Balanced Equation: 2H2 + O2 → 2H2O
- The limiting reactant is hydrogen, as it runs out first based on the stoichiometric ratio in the balanced equation.
- Calculated Theoretical Yield: From 3 moles of H2, we can yield 3 moles of H2O (theoretical yield = 6 grams of water).
By practicing with these kinds of examples, you can gain a practical understanding of the theoretical yield concept and its applications in various chemical reactions.
Conclusion: Theoretical Yield and Its Significance
Theoretical yield remains a cornerstone concept in chemistry, guiding effective practices from academic study to practical laboratory applications. Understanding how to accurately calculate and interpret theoretical yield can significantly impact the efficiency and success of chemical reactions, paving the way for advancements in research and industrial processes. As we move forward into 2025, mastering these fundamental skills will be pivotal for upcoming chemists and industry professionals alike.
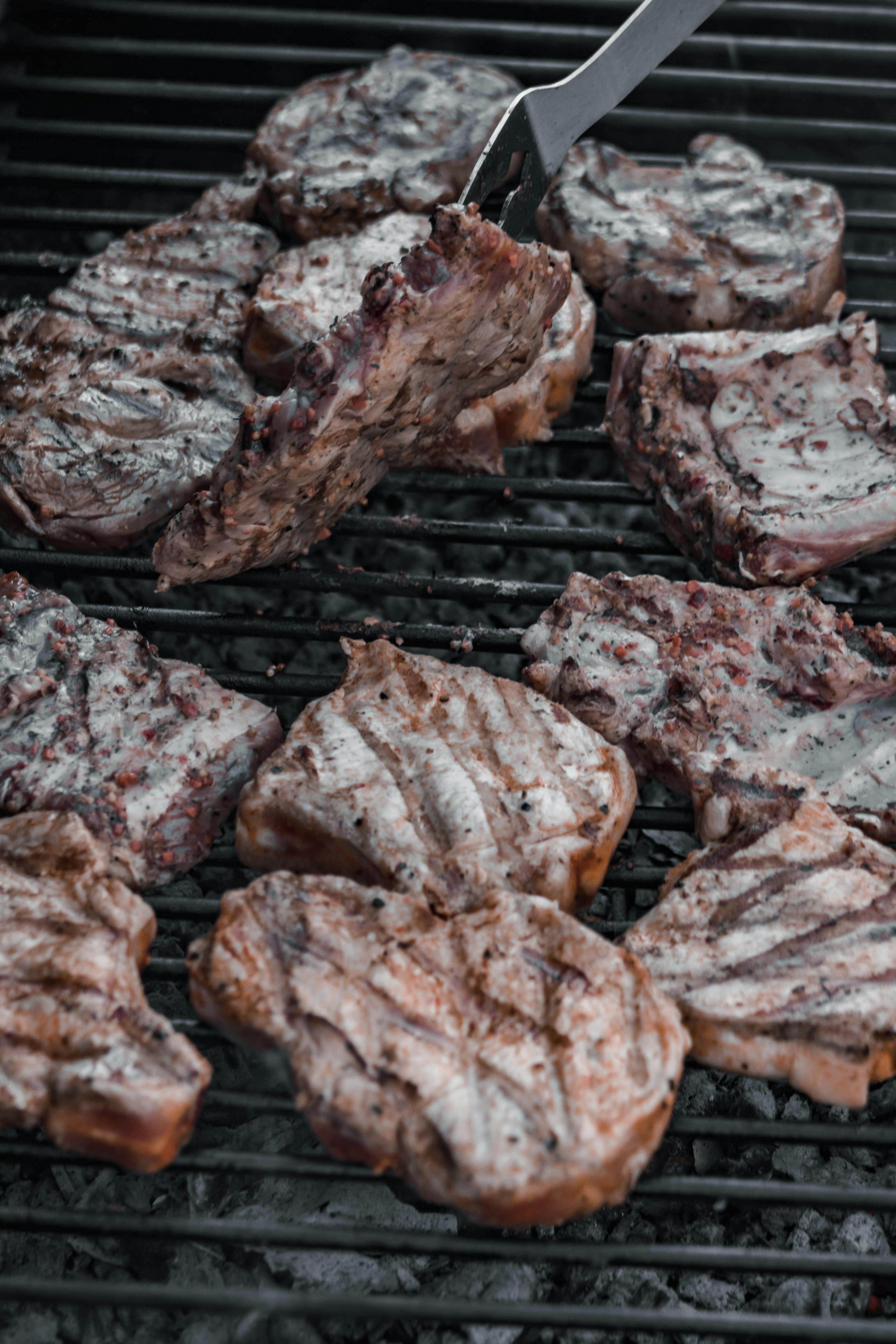
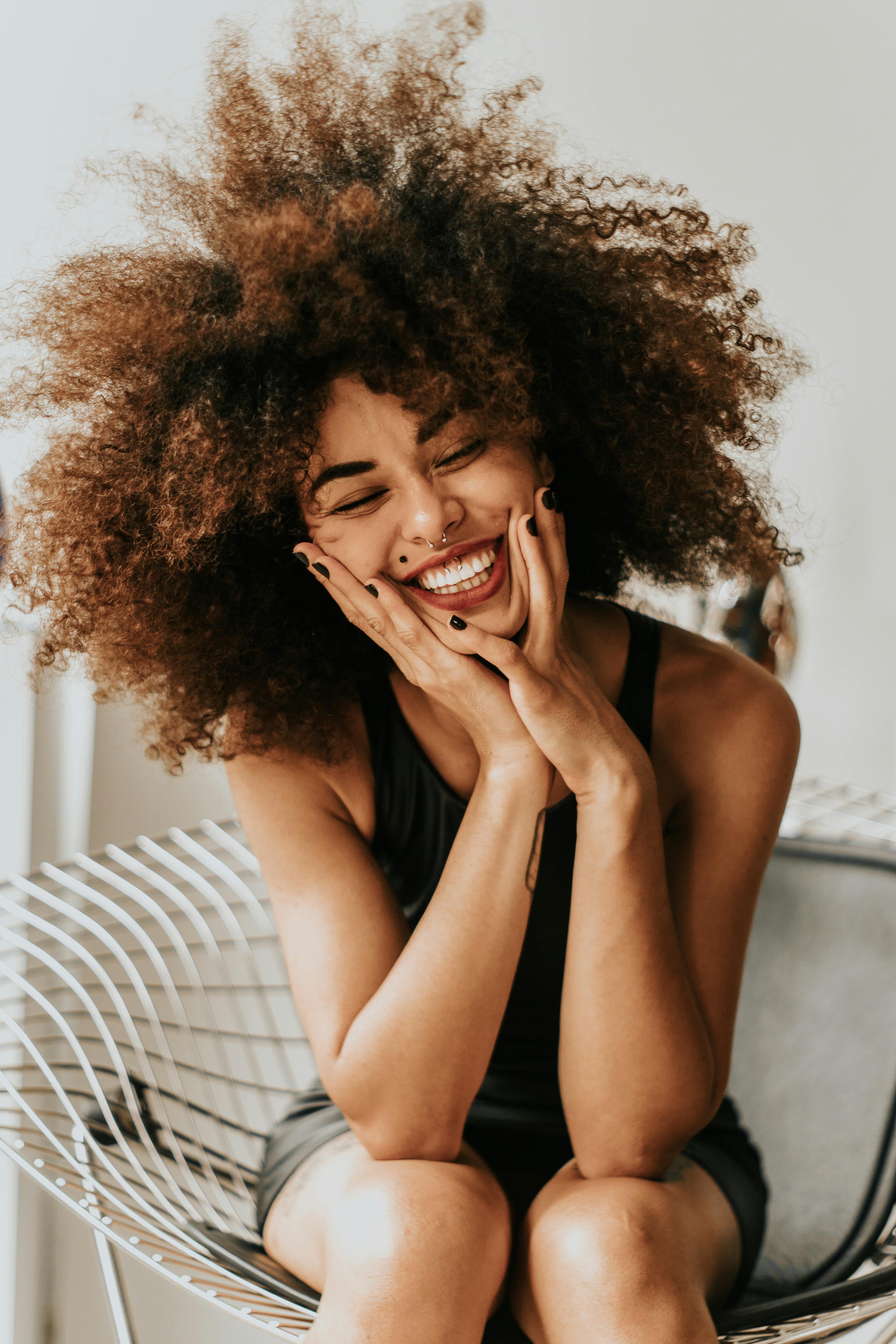